Photo-energy conversion for renewable energy production
In this topical axis we are studying molecular systems for renewable energy production. Ultrafast transient absorption and fluorescence spectroscopy is applied to decipher the light-induced ultrafast processes involved in either dye-sensitized solar cells (DSSCs) or in molecular dyads made of iron and cobalt for reduction of protons leading to hydrogen production through photo-catalysis.
Quasi-transparent near-infrared absorbing DSSCs are studied within the French network VISION-NIR led by F. Sauvage (LCRS, Amiens) in collaboration with F. Odobel and Y. Pellegrin (CEISAM, Nantes). Our recent results are described below.
Within the French-German project Sun-Hy led by C. Cebriàn (ICS, Strasbourg) and in collaboration with the group of M. Bauer (U Paderborn) we aim at investigating ultrafast charge transfer processes between Fe photo-sensitizers and Co catalysts. In addition to our UV/VIS femtosecond spectroscopy, the group of K. Heyne (FU Berlin) applies ultrafast vibrational spectroscopy to these systems. See details below.
Ultrafast Spectroscopy of Solar Cells
We are studying the photo-induced molecular process in so-called dye-sensitized solar cells (DSSCs), which hold great promise for diversified applications in photo-voltaics, i.e. alternative to roof-top panels made of Si. Imagine we would use our windows for producing electricity: Impossible with opaque solar panels, but possible with transparent solar cells made with molecules. Indeed, in DSSCs, the absorption of the dyes can be optimised for the near-IR spectral range, rendering the cells almost completely transparent in the VIS part of the spectrum (figure 1). Within the national project VISION-NIR, we pursued this strategy and achieved record performances of our prototype cells in terms of average visible transmittance (AVT) and power conversion efficiency (PCE) [1,2].
What does ultrafast spectroscopy of DSSCs provide in this context? The photo-induced processes in DSSCs occur on time scales of 0.2 to 100 ps. The main processes studied are depicted in figure 2: After light absorption, the near-IR molecules release electrons, which are injected into the TiO2 semiconductor, where they make up the current density that flows between the photo-anode and the cathode. Ultrafast spectroscopy measures this process directly via the time-dependent formation of the dye’s cation absorption, and we can evaluate its efficiency. However, a detrimental process is in competition with the carrier injection, and this is energy transfer to the low energy states of molecular aggregates [1,2]. Our spectroscopy shows how this process can be minimized, but it is still the main limiting factor in the near-IR DSSCs [3].
The next development is to conceive near-IR dyes with high enough oxidation potential so that the aggregates can contribute to the electron injection and thus to the photo-current of the cells. This will be tested directly by ultrafast transient absorption spectroscopy.
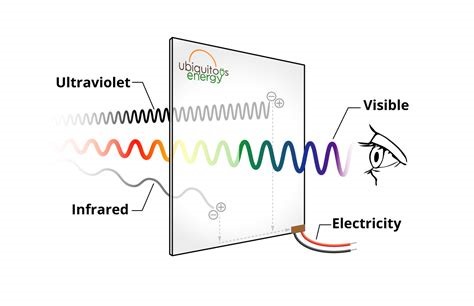
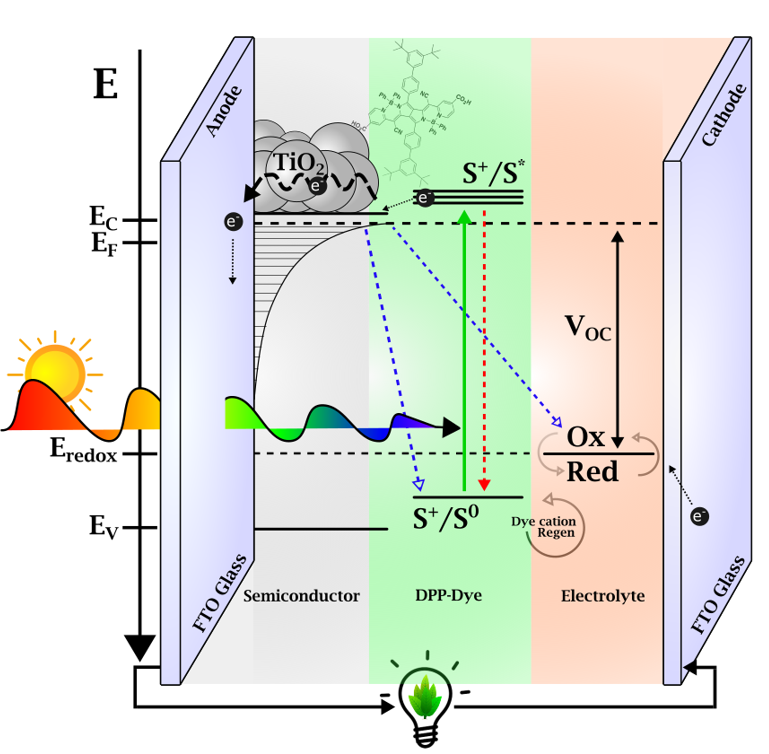
Towards a Greener Hydrogen Production using Abundant Metals
Within the quest of improved technologies for renewable energy production, Hydrogen (H2) is a very promising energy vector. It finds applications in many sectors, such as transportation, industry or energy production and storage, and its combustion does only produce water. Yet, the way it is produced nowadays is not environment-friendly enough, in terms of CO2 balance among other factors. The general trend is to promote H2 production by water splitting, or electrolysis, with electricity produced by renewable and CO2-less techniques (“green hydrogen”).
One step further could be to perform water splitting thanks to photocatalysis, i.e. the direct use of sunlight as energy source, instead of electrolysis, in a photo-electrochemical cell (figure 3). At present, this approach is still under development and uses metal-based photosensitisers, i.e. light-harvesting molecules, and photocatalysts, to reduce protons into hydrogen. The most efficient photosensitizers contain rare metals, such as Ruthenium (Ru), Platinum (Pt), Palladium (Pd), or Iridium (Ir), and are thus extremely expensive and absolutely not viable for an industry scale production [3]. One solution could then be the use of Earth-crust abundant metals like Iron (Fe), Copper (Cu) and Cobalt (Co).
When combined in a single dyad, photo-active metal complexes are made of three different parts: (i) a light-harvesting iron-based complex absorbs photons and generates electrons that travel in the molecule through a (ii) bridging ligand to the (iii) cobalt-based catalyst that reduces water into H2 (figure 3). Sensitizers based on organic molecules are an alternative, but the most common proton reduction catalysts are based on Co [4].
BioDyn is part of the international project SunHy (Photocatalysts based on Earth-abundant metals: towards a sustainable production of solar hydrogen) that gathers French and German partners in order to move research forward in the domain of water photocatalysis and green hydrogen production with metal-based complexes. The team offers the SunHy consortium its expertise in ultrafast spectroscopy to probe the electron transfer reactions within the photosensitizers [5,6] and assess the lifetimes of the different molecular states. This probing is performed using Transient Absorption Spectroscopy (TAS) that allows a deep insight of the electron transfer dynamics in the molecules thanks to its sub-ps time resolution. Ultrafast fluorescence detected by up-conversion or the streak camera technique is a complementary approach to probe the conversion of the excited photo-sensitizer molecule into the cationic form.
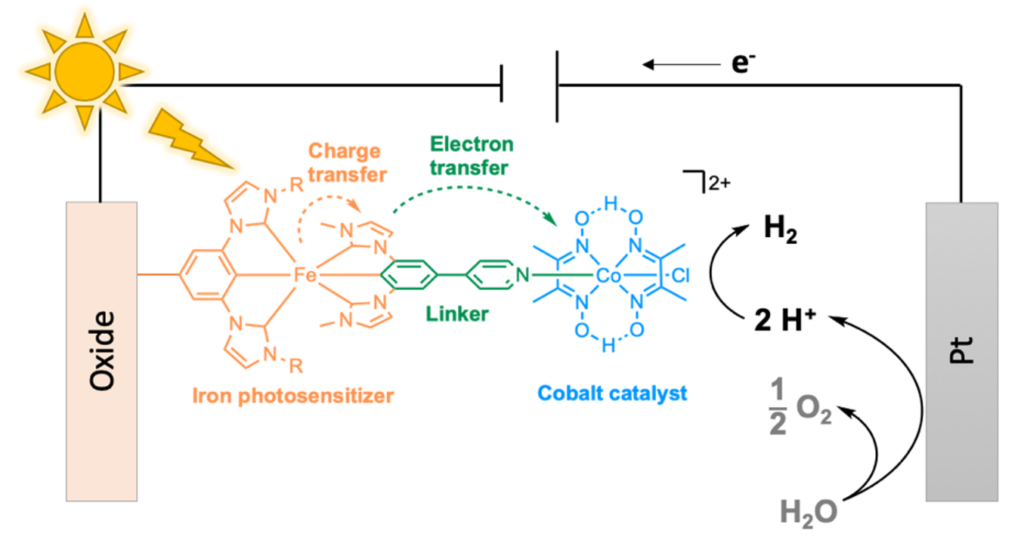
References
[1] W. Naim, et al., J. Am. Chem. Soc. Au, 2021, 1(4), 409-426, https://doi.org/10.1021/jacsau.1c00045
[2] T. Baron, et al., Angew. Chem., 2022, 134, e202207459, https://doi.org/10.1002/anie.202207459
[3] I. Nikolinakos, PhD thesis University of Strasbourg (2022), https://theses.hal.science/tel-03971579/document
[4] P. Dierks, et al (2022) Inorg. Chem. Front., 9, 206-220, https://doi.org/10.1039/D1QI01112J
[5] C. D. Windle et al., JACS, (2019) 141, 9593 https://doi.org/10.1021/jacs.9b02521
[6] C. Cebrián et al., (2022), PCCP, https://doi.org/10.1002/cphc.202100659