Transient Absorption Spectroscopy
In a Transient Absorption Spectroscopy (TAS) set-up, a sample containing a species – molecule in solution or in film in our case – is placed at the focus of two different beams called pump and probe. The role of the pump beam is to excite the electrons of the different species. The probe beam is used to measure the absorption spectrum of the “transient” population in the excited states. After a certain amount of time (from femtoseconds to milliseconds) these excited states react or relax to form new species called photoproducts. To monitor the evolution of the spectroscopic signatures of the transient species, the probe beam is delayed compared to the pump (figure 1). TAS is therefore a dynamic measurement that allows one to probe the electronic excited state relaxation and reaction dynamics taking place inside a molecule.
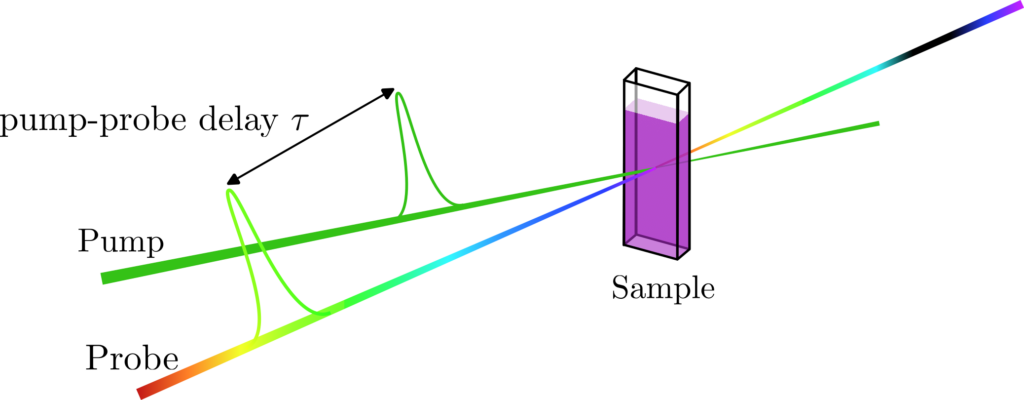
The Transient Absorption Spectroscopy setup built by the BioDyn team allows one to probe ultrafast photoreaction dynamics with a 60 fs time resolution. Combined with a very broad range of excitation wavelengths – from 300 nm up to 1500 nm, using a TOPAS (Light Conversion) for the pump pulse – this setup is very often needed in various projects such as Retinal Proteins, Ultrafast bio-mimetic photo-switches and molecular motors, Iron Complexes for photocatalysis of Hydrogen, or Dye-Sensitised Solar Cells, to probe the very processes at work and better understand them.
Fluorescence Up-conversion spectroscopy (FLUPS)
The fluorescence up-conversion technique is based on sum frequency generation (SFG): the detected signal is the sum of the emitted fluorescence (frequency ωf, wave vector kf) with a 60 fs gate beam (ωg, kg). The latter is used to sample the fluorescence signal at different time delays between excitation and gate pulses. The SFG occurs between the spatially and temporally superimposed beams in a non-linear optical medium i.e. a BBO crystal. Thus, an up-converted signal (ωsum ,ksum) is generated. The process fulfills energy conservation and phase matching conditions as expressed by:
ωsum = ωf + ωg and ksum = kf + kg
With our home-built up-conversion setup, we perform time-resolved fluorescence spectroscopy with ~150 fs time resolution and a broadband spectral detection enabled by type II frequency mixing (figure 2). The pump and the gate pulses are produced at a 5kHz repetition rate by an optical parametric amplifier (OPA) pumped by the fundamental beam of a Titanium:Sapphire (Ti:Sa) regenerative amplifier with central wavelength 800 nm. Subsequent 2nd or 4th harmonic generation offers a spectral tunability from 260 nm to the near IR. Originally, we had rather implemented down-conversion, by difference frequency mixing, in order to convert UV fluorescence into the visible spectral range for efficient detection with a Si-based CCD camera. [1][2]

Fluorescence up- or down-conversion spectroscopy (FLUPS) is complementary to transient absorption spectrscopy (TAS). While the interpretation of TAS is very often obscured by the spectral overlap of the signatures of different electronic states (ground state, excited states, and photoproduct), FLUPS monitors only the signature of the molecular excited states. We use FLUPS to investigate different photophysical processes such as the ultrafast Photo-isomerisation process in Retinal proteins (figure 3) or the mechanism of ultrafast energy transfer between chromophores in dye-loaded nanoparticles. In the latter case, we implement polarization-resolved fluorescence detection, which enables to measure the fluorescence anisotropy decay at a femtosecond time scale.

Streak camera
The streak camera set-up allows for direct spectrally and time-resolved measurements of fluorescence/phosphorescence in the range of 250 to 850 nm. Unlike fluorescence up-conversion, it is an extremely sensitive method when operated in the single photon counting mode, but its time resolution is limited to 10 ps. The excitation beam is produced by Tangerine, Amplitude Yb:fiber Amplifier system, 0.3 ps pulse duration, in conjunction with a home-built Optical Parametric Amplifier (OPA), which allows tuning the excitation wavelength from 520 nm to 900 nm (+ frequency doubling) and the repetition rate from 1Hz to 20MHz. The collimated excitation beam is focused in the sample (solution or films) using a microscope objective. The emitted fluorescence is collected and focused on the entrance slit of the detection stage. The detection stage comprises a monochromator in conjunction with a streak camera (Hamamatsu streak scope C10627). The latter one offers different time ranges for detection from one nanosecond to milliseconds with a time resolution down to 10 ps. Furthermore, a confocal microscope for the spatial selection of the fluorescence and an imaging system that allows fluorescence spot characterization are integrated in the experimental setup.
Due to is broad spectral coverage, and high time resolution, the streak camera setup is extensively used to study systems with competitive photo processes that occur at different time scales such as fluorescence and phosphorescence in metal complexes (figure 4) or energy transfer and singlet-singlet annihilation in organic nanoparticles.

References
[1] Jérémie Léonard, T Gelot, K Torgasin, Stefan Haacke. Ultrafast fluorescence spectroscopy of biologically relevant chromophores using type II difference frequency generation. Journal of Physics: Conference Series, 2011, 277, ⟨10.1088/1742-6596/277/1/012017⟩. ⟨hal-02465495⟩
[2] Gelot, Thomas, Patricia Tourón-Touceda, Olivier Crégut, Jérémie Léonard, et Stefan Haacke. « Ultrafast Site-Specific Fluorescence Quenching of 2-Aminopurine in a DNA Hairpin Studied by Femtosecond Down-Conversion ». The Journal of Physical Chemistry A 116, no 11 (22 mars 2012): 2819 25. https://doi.org/10.1021/jp212187m.