Les matériaux magnétoélectriques, grâce au couplage existant entre leurs propriétés magnétiques et électriques, permettent la manipulation de l’aimantation par un champ électrique. Ces matériaux suscitent actuellement un intérêt considérable car ils ouvrent de nouvelles perspectives en termes de dispositifs de mémoire. En effet, une mémoire magnétoélectrique combinerait le meilleur des deux mondes que sont les FeRAMs et les MRAMs. A l’heure actuelle, le développement des mémoires magnétoélectriques semble être limité par la rareté des matériaux magnétoélectriques et encore plus par la rareté des matériaux magnétoélectriques présentant un ordre magnétique à température ambiante.
• Le ferrite de gallium Ga2-xFexO3 comme matériau alternatif pour la manipulation magnétoélectrique de l’aimantation.
La manipulation magnétoélectrique de la magnétisation a été expérimentalement démontrée à température ambiante avec un antiferromagnétique ferroélectrique, le BiFeO3 (BFO), seul matériau considéré jusqu’à présent dans la littérature présentant à la fois un couplage magnétoélectrique et un ordre magnétique à température ambiante. Cependant, l’ordre magnétique étant antiferromagnétique, l’utilisation effective du BFO nécessite l’emploi d’une couche supplémentaire à laquelle il est magnétiquement couplé. Cela rend la fabrication des dispositifs plus compliquée et le dispositif lui-même sujet à des défaillances. Il est donc essentiel de faire des efforts pour développer des matériaux magnétoélectriques présentant une aimantation non nulle à température ambiante. Dans cette optique, nous avons considéré avec grand intérêt la ferrite de gallium Ga2-xFexO3 (0.8 < x < 1.4) (GFO). Il apparaît en effet comme le matériau alternatif parfait au BFO dans les mémoires magnétoélectriques : il est ferrimagnétique au-dessus de la température ambiante pour x=1.4 et pyroélectrique avec un fort couplage magnétoélectrique.
![]() |
---|
Figure 1 : Crystallographic structure of GFO together with its net electric polarization (P) and magnetization (M) directions. GFO adopts an orthorhombic structure, crystallizing in the space group Pc21n with a = 0.87512 ± 0.00008 nm, b = 0.93993 ± 0.00003 nm and c = 0.50806 ± 0.00002 nm. The Ga3+ and Fe3+ cations are distributed on four types of sites labeled Fe1, Fe2, Ga1 and Ga2. |
• Films minces multiferroïques à température ambiante
avec une faible polarisation cependant, par rapport à ce qui est attendu (0.2 vs. 25 µC/cm2)
![]() |
---|
Figure 2 : Ferroelectric loop measured on a GFO1.4 thin film – The polarization is about 0.2 µC/cm2, i.e. a hundred times smaller than expected [Thomasson et al. J. Appl. Phys. 113, 214101 (2013)] |
• Un matériau à la polarisation électrique robuste
La polarisation des films a été déterminée à la fois par la méthode non destructive de la diffraction résonante des rayons X et par la microscopie électronique à haute resolution.
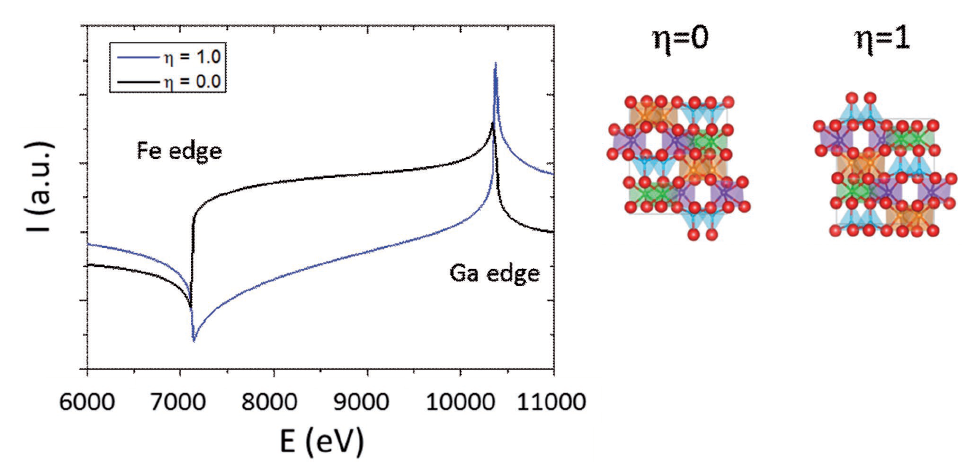
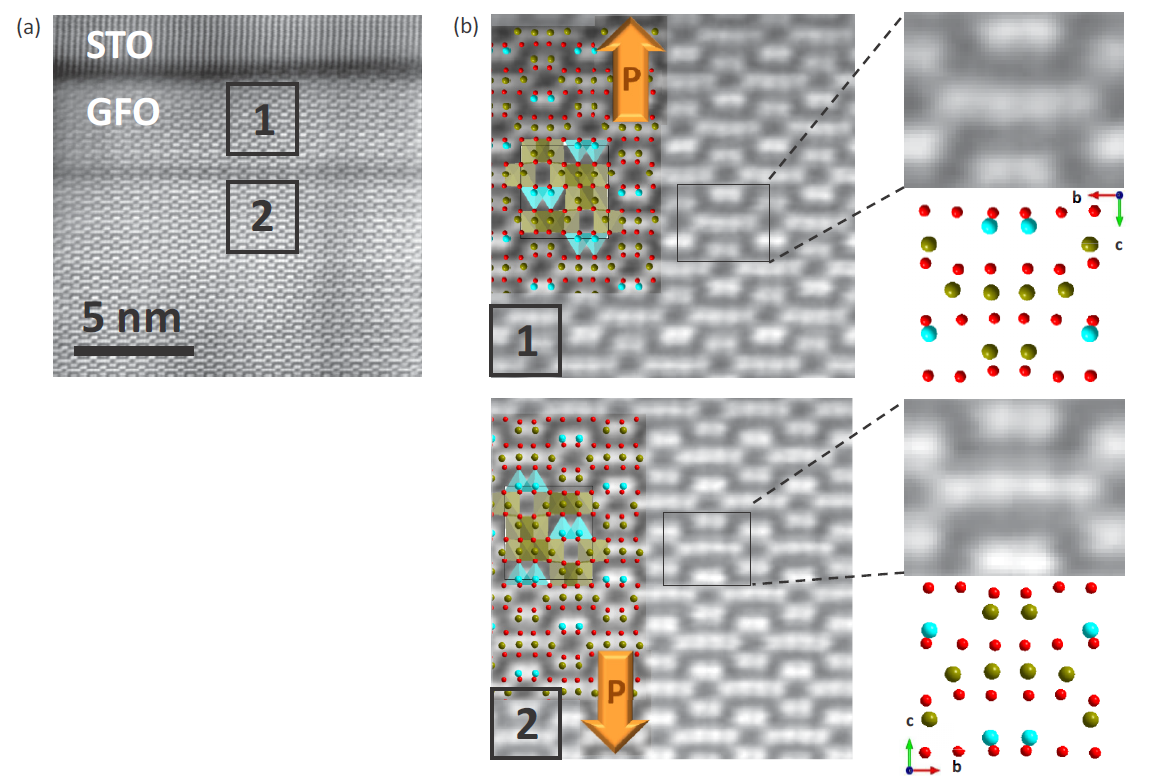
• Un comportement ferrimagnétique complexe
avec un moment orbital magnétique non nul et fortement anisotrope – Indication d’un fort couplage magnétoélectrique.
![]() |
---|
Figure 4 : XMCD spectra measured on a GFO1.4 thin film in grazing (GI) and normal (NI) incidence. The opposite sign of the integrated signals evidences an important anisotropy of the orbital moment [Preziosi et al. Phys. Rev. B 103, 184420 (2021)] |
Nous travaillons actuellement sur un projet visant à metre à profit les propriétés magnétoélectriques de GFO pour moduler le retournement de l’aimantation dans des dispositifs à effet Hall de spin. (projet ANR “MISSION”).
Read more in:
[1] | Trassin, M.; Viart, N.; Versini, G.; Loison, J. L.; Vola, J. P.; Schmerber, G.; Cregut, O.; Barre, S.; Pourroy, G.; Lee, J. H.; Jo, W.; Meny, C. Epitaxial Thin Films of Multiferroic GaFeO3 on Conducting Indium Tin Oxide (001) Buffered Yttrium-Stabilized Zirconia (001) by Pulsed Laser Deposition. Appl. Phys. Lett. 2007, 91 (20), 2504–2504. https://doi.org/10.1063/1.2813020 |
[2] | Trassin, M.; Viart, N.; Versini, G.; Barre, S.; Pourroy, G.; Lee, J.; Jo, W.; Dumesnil, K.; Dufour, C.; Robert, S. Room Temperature Ferrimagnetic Thin Films of the Magnetoelectric Ga2-xFexO3. J. Mater. Chem. 2009, 19 (46), 8876–8880. https://doi.org/10.1039/b913359c. |
[3] | Lefevre, C.; Shin, R. H.; Lee, J. H.; Oh, S. H.; Roulland, F.; Thomasson, A.; Autissier, E.; Meny, C.; Jo, W.; Viart, N. Reduced Leakage Currents and Possible Charge Carriers Tuning in Mg-Doped Ga0.6Fe1.4O3 Thin Films. Appl. Phys. Lett. 2012, 100 (26), 262904. https://doi.org/10.1063/1.4729872. |
[4] | Thomasson, A.; Cherifi, S.; Lefevre, C.; Roulland, F.; Gautier, B.; Albertini, D.; Meny, C.; Viart, N. Room Temperature Multiferroicity in Ga0.6Fe1.4O3:Mg Thin Films. J. Appl. Phys. 2013, 113 (21), 214101. https://doi.org/10.1063/1.4808349.. |
[5] | Thomasson, A.; Ibrahim, F.; Lefevre, C.; Autissier, E.; Roulland, F.; Mény, C.; Leuvrey, C.; Choi, S. G.; Jo, W.; Crégut, O.; Versini, G.; Barre, S.; Alouani, M.; Viart, N. Effects of Iron Concentration and Cationic Site Disorder on the Optical Properties of Magnetoelectric Gallium Ferrite Thin Films. RSC Adv. 2013, 3, 3124. https://doi.org/10.1039/C2RA22681B.. |
[6] | Thomasson, A.; Kreisel, J.; Lefevre, C.; Roulland, F.; Versini, G.; Barre, S.; Viart, N. Raman Scattering of Magnetoelectric Gallium Ferrite Thin Films. J. Phys.-Condens. Matter 2013, 25 (4). https://doi.org/10.1088/0953-8984/25/4/045401.. |
[7] | Lefevre, C.; Roulland, F.; Thomasson, A.; Meny, C.; Porcher, F.; Andre, G.; Viart, N. Magnetic and Polar Properties’ Optimization in the Magnetoelectric Ga2-xFexO3 Compounds. J. Phys. Chem. C 2013, 117 (28), 14832–14839. https://doi.org/10.1021/jp403733b.. |
[8] | Kundys, B.; Roulland, F.; Lefevre, C.; Meny, C.; Thomasson, A.; Viart, N. Room Temperature Polarization in the Ferrimagnetic Ga2-XFexO3 Ceramics. J. Eur. Ceram. Soc. 2015, 35 (8), 2277–2281. https://doi.org/10.1016/j.jeurceramsoc.2015.02.029.. |
[9] | Demchenko, A.; Chang, Y.; Chikoidze, E.; Berini, B.; Lefevre, C.; Roulland, F.; Ulhaq-Bouillet, C.; Versini, G.; Barre, S.; Leuvrey, C.; Favre-Nicolin, V.; Boudet, N.; Zafeiratos, S.; Dumont, Y.; Viart, N. Tuning the Conductivity Type in a Room Temperature Magnetic Oxide: Ni-Doped Ga0.6Fe1.4O3 Thin Films. RSC Adv. 2016, 6 (34), 28248–28256. https://doi.org/10.1039/c6ra01540a.. |
[10] | Lefevre, C.; Thomasson, A.; Roulland, F.; Favre-Nicolin, V.; Joly, Y.; Wakabayashi, Y.; Versini, G.; Barre, S.; Leuvrey, C.; Demchenko, A.; Boudet, N.; Viart, N. Determination of the Cationic Distribution in Oxidic Thin Films by Resonant X-Ray Diffraction: The Magnetoelectric Compound Ga2-xFexO3. J. Appl. Crystallogr. 2016, 49 (4), 1308–1314. https://doi.org/10.1107/S1600576716010001.. |
[11] | Lefevre, C.; Demchenko, A.; Bouillet, C.; Luysberg, M.; Devaux, X.; Roulland, F.; Versini, G.; Barre, S.; Wakabayashi, Y.; Boudet, N.; Leuvrey, C.; Acosta, M.; Meny, C.; Martin, E.; Grenier, S.; Favre-Nicolin, V.; Viart, N. Nondestructive Method for the Determination of the Electric Polarization Orientation in Thin Films: Illustration on Gallium Ferrite Thin Films. Small Methods 2017, 1, 1700234. https://doi.org/10.1002/smtd.201700234.. |
[12] | Homkar, S.; Preziosi, D.; Devaux, X.; Bouillet, C.; Nordlander, J.; Trassin, M.; Roulland, F.; Lefèvre, C.; Versini, G.; Barre, S.; Leuvrey, C.; Lenertz, M.; Fiebig, M.; Pourroy, G.; Viart, N. Ultrathin Regime Growth of Atomically Flat Multiferroic Gallium Ferrite Films with Perpendicular Magnetic Anisotropy. Phys. Rev. Mater. 2019, 3 (12), 124416. https://doi.org/10.1103/PhysRevMaterials.3.124416.. |
[13] | Preziosi, D.; Homkar, S.; Lefevre, C.; Salluzzo, M.; Viart Unusual Anisotropic Magnetic Orbital Moment Obtained from X-Ray Magnetic Circular Dichroism in a Multiferroic Oxide System. Phys. Rev. B 2021, 103 (18), 184420. https://doi.org/10.1103/PhysRevB.103.184420.. |