Nuclear Spin Qudits for quantum information processing
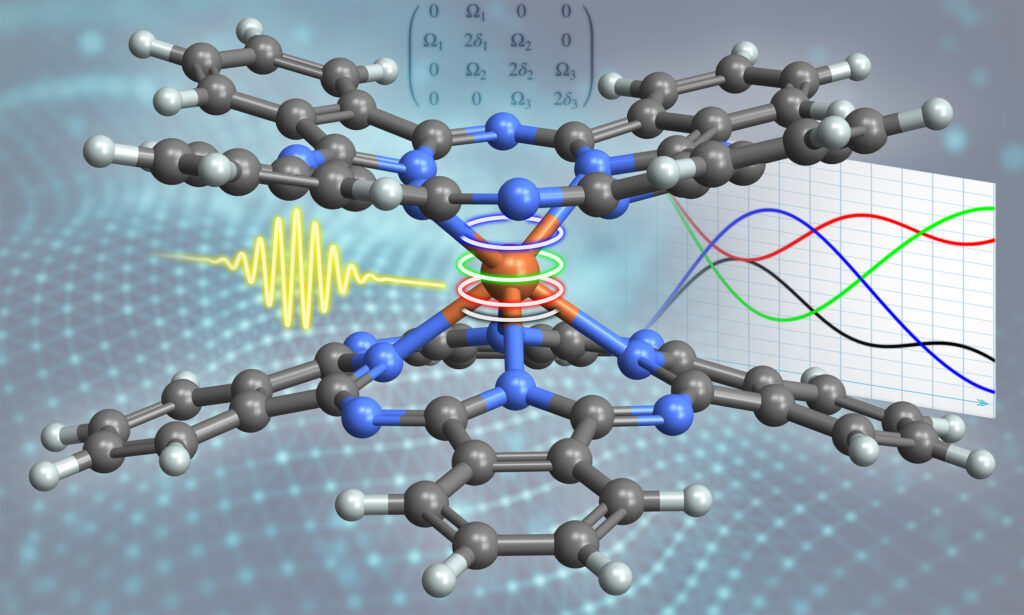
This multidisciplinary project combines expertise from nuclear, atomic and solid-state physics, along with materials chemistry. It addresses a fundamental issue concerning the possibility of modifying and eventually controlling the hyperfine interactions which play a key role in single-molecule magnets (SMM). As an extension of the well-known two-level quantum bits (qubits), multilevel systems called qudits (where d represents the Hilbert space dimension) have been suggested to reduce the number of iterations in quantum computation algorithms. This has been tested experimentally in SMM where multilevels are originated from the nuclear spins and the associated hyperfine interactions. Controlling or modifying these interactions may open the way to the manipulation of the multilevel system’s properties, thus leading to improvements or elaboration of new quantum computation algorithms. In close collaboration with experimental chemists and physicists from the Karlsruhe Institute of Technology (Professors Mario Ruben and Wolfgang Wernsdorfer) we propose to establish a theoretical framework to answer this issue.
This project is part of the transnational QUSTEC program.
Publications
- Noisy Qudit vs Multiple Qubits : Conditions on Gate Efficiency. Npj Quantum Information (2024). https://arxiv.org/abs/2302.04543
Loschmidt echo and quantum fidelity
In a famous controversy with Ludwig Boltzmann at the dawn of modern statistical mechanics, Joseph Loschmidt pointed out that, if one reverses the velocities of all particles in a physical system, the latter would evolve back to its initial state, thus violating the second law of thermodynamics. The main objection to this line of reasoning is that velocity reversal is an extremely unstable operation and that tiny errors would quickly restore “normal” entropy increase. Nevertheless, time reversal is indeed possible, as was shown in spin echo experiments performed since the 1950s. Loschmidt’s idea has recently experienced a resurgence of interest in the context of quantum information theory. In order to estimate the robustness of a physical system, the following procedure has been suggested: a single quantum particle evolves under the action of a chaotic Hamiltonian H0 until a time T; then, it is evolved backwards in time until 2T with the original Hamiltonian plus a small perturbation (the “environment”). The square of the scalar product of the initial and final states defines the Loschmidt echo or fidelity of the system, and is a reasonable measure of its quantum coherence. Theoretical and numerical studies showed that the Loschmidt echo decays exponentially with the time delay T.
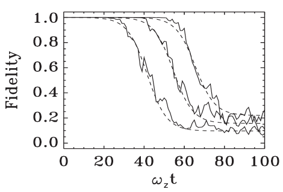
What happens when one deals, not with a single particle, but with a large system of interacting particles, such as the electrons in a quantum well or a Bose-Einstein condensate confined in a magnetic trap? We have simulated numerically the evolution of the quantum fidelity for such many-body systems and the results were intriguing: the fidelity does not decay exponentially, but rather stays close to unity until a critical time, after which it drops abruptly. This unusual behavior is related to the fact that, for a many-body system in the mean-field approximation, the Hamiltonian depends on the wave function itself. This effect seems to be a generic feature of interacting many-particle systems.
Publications
- Fidelity Decay in Trapped Bose-Einstein Condensates. Phys. Rev. Lett. 100, 050405 (2008). https://doi.org/10.1103/PhysRevLett.100.050405
- Loschmidt Echo in a System of Interacting Electrons. Phys. Rev. Lett. 97, 190404 (2006). https://doi-org.scd-rproxy.u-strasbg.fr/10.1103/PhysRevLett.97.190404